On my last day in the Blue Mountains, the cloud lifted and I was able to see the spectacular views which have made the area famous. I spent a few hours walking along the cliff top from Leura to Katoomba, with a diversion along the road where an area of track was damaged. In theory, the whole walk is supposed to take two days, but I did about half the walk in a few hours. There are plenty of steps going up and down – inevitable in the Blue Mountains terrain – but it was a fairly gentle walk overall. I had more opportunity to photograph the plants than on the previous day, when it was often too wet for me to use my camera.
I had no hope of identifying many of the plants I saw. Although Australia’s plants are incredibly diverse, and the Blue Mountains is a biodiversity hotspot, without flowers many of the plants appear very similar. With every step, I spotted small shrubs with short, narrow leaves, grey-green, leathery and with a sharp leaf-tip. For New Zealanders, think of something like a mānuka plant. Sometimes the leaves were wider or narrower, sometimes longer or shorter, more or less spiky, greener or greyer or even reddish. Sometimes the plants were low to the ground and sometimes they were almost tree-sized. But there was always a certain texture to the leaves, a toughness which means they don’t offer much of a mouthful to a hungry animal, nor do they wilt under the merciless Australian sun.
To someone trying to make sense of the landscape, it may seem logical to classify these plants together. They have many features in common and as a group they are easy to identify, even if they are hard to tell apart from one another. And there is a collective name for this type of plant – sclerophyll, which comes from the ancient Greek words for ‘hard’ and ‘leaf’.
But when these plants flower, they don’t seem quite so similar to one another. In fact, many of them are very different indeed. The reason they seem similar without flowers is that they are all using a similar strategy to survive, conserving water with their small, tough leaves. The evolution of a diverse group of plants has converged on this particular set of characteristics.
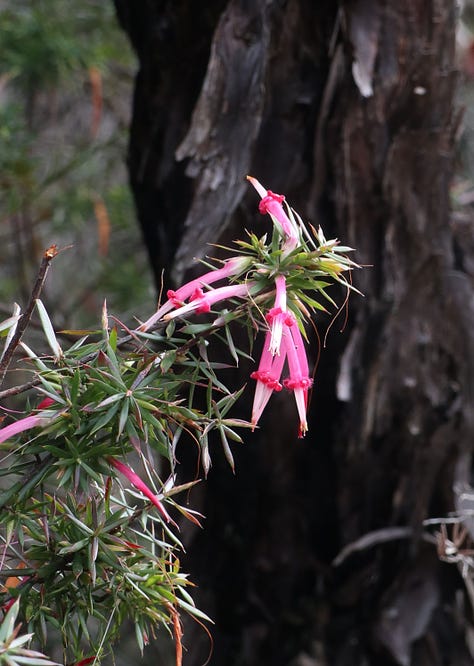
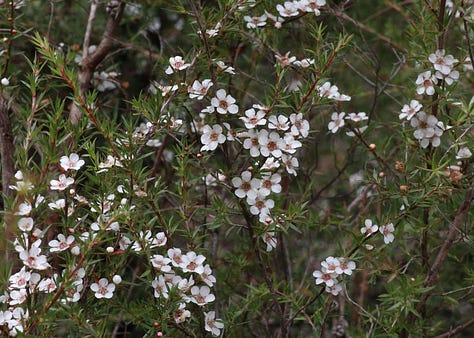
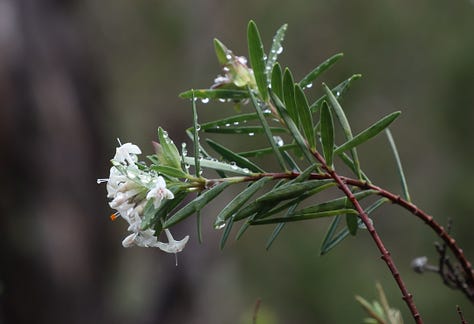
Botanists don’t necessarily classify plants by their most obvious features. As I mentioned a few weeks back, Karl Linnaeus came up with a system based mostly on flower features. What he didn’t know at the time, because he lived a century before Darwin and Wallace, was that his system would prove to be remarkably good at representing the evolution of plants. On the whole, he had classified species together which had evolved from common ancestors – a family tree or whakapapa for plants. It wasn’t perfect, but it was a great start.
Since then, botanists have striven to make their classification match the evolution of the different plant groups as closely as possible. Until the last few decades, they had to infer this by looking at the appearance of the plants, or sometimes by analysing chemical compounds within the plants. It was crucial that they used the right set of features in their classification, but they were sometimes led astray by superficially similar features which didn’t come from common ancestry.
The last thirty years have seen a revolution, though. Now, we can look at a plant’s genetic code. It’s like looking at photographs when we’ve previously only had drawings or paintings. I will write about this revolution, but before I do that, I want to explain why it’s important. Why does it matter whether one shrub with tough little leaves is related to gum trees and another related to rhododendron?
There’s a simple answer to the question: because it matters to other living things. If we only wanted to understand plants from a human perspective, then it wouldn’t matter. We could classify plants by their uses, or by flower colour, or by the texture of their leaves. But biologists want to understand how the natural world works and how living things interact with each other. And it turns out that ancestry, or evolutionary history, is fundamental.
Much of my work over the last 20 years has been about trying to predict which insect pests and plant diseases overseas could affect the plants growing in New Zealand, both native and introduced. This is an inexact science, at best. Sometimes, it’s little more than an educated guess. But understanding how plants are related to each other is one of the most important clues.
Biological control, such as the programme which New Zealand has for managing weeds, is one of the best examples. The first New Zealand attempt at biological control – introducing stoats, ferrets and weasels to control rabbits – was an unmitigated disaster. Rabbits were not controlled and many native birds were driven to extinction or only survived on predator-free islands. Even back in the 1870s this was entirely predictable. The problem was not our ability to predict what stoats, ferrets and weasels would eat. The problem was that the predictions were ignored.
We’ve made some progress since then, and potential biological control agents undergo a rigorous testing and assessment programme. An animal such as a stoat would never be considered, because it kills many different types of animal. It would be like releasing a herd of goats into a bush reserve to control the weeds – it’s pretty clear that the goats aren’t going to confine themselves to eating only the plants we want them to eat and leaving everything else.
But many animals are much more discriminating. Staying with Australia and mammals – the koala mostly confines itself to just a few species of gum tree, although it does sometimes munch on other plants. Insects, which are far more likely to be used for biological control these days, are similar. Some are the equivalent of goats and eat just about anything – the desert locust for example. But many are more like the koala.
Researchers have developed a way to test this. The method was originally developed by an Australian entomologist, Anthony Wapshere, who was looking for insects to use for controlling weeds. He published his method in 1975, and while it has since been updated, the central idea remains the same.
Wapshere was looking for insects which would feed on only a limited range of plants – ideally, just the weed he wanted to control. His idea was to test his candidate insects on the plants most closely related to the weed he was targeting. His method did include some other plants as well, but the most closely related plants were the most important.
The botanical group which gets the most attention in Wapshere’s method, and is also one of the most important to botanists, is the botanical family. Of course, family has an everyday English usage, but in botany, it has a precise meaning. The scientific naming method invented by Linnaeus has two parts – genus and species. An example I gave in a recent article is Coprosma foetidissima, where Coprosma (meaning poo-smelling) is the genus and foetidissima (the stinkiest) is the species. Close relatives, such as Coprosma repens, belong to the same genus but a different species.
The botanical classification of family is one level up from the genus. In the case of Coprosma, the family name is Rubiaceae, named for a plant called Rubia which was used as a red dye (ruber means red in Latin). However, there’s a more interesting plant (at least in my opinion) which belongs to this family – the coffee plant. So, sometimes people talk about this family as the “coffee family”.
Since Wapshere’s method was adopted, there have been very few surprises among the insects introduced to control weeds. Almost all of those surprises have been cases where the insects fed on members of the same botanical family as the target species. Sometimes there are a lot of species in a particular family, and not every species gets tested. This may or may not be a problem, depending on how important the untested plants are. Or, rather, it depends who you ask, because we all have different perceptions on how important particular plants are.
One of the recent species targeted for biological control in New Zealand is tradescantia, the former houseplant which is now the scourge of people working to restore native forest. I can remember it being discussed as a possible species for biological control 20 years ago, and it was an ideal candidate, botanically speaking. It belongs to a family which is mostly tropical. New Zealand has no native species belonging to the family, nor does the family include any crops. In climates such as New Zealand’s, there are a couple of garden plants and a fair few house plants, but nothing of major value. So even if there were a few plants in the same family which were eaten by a biological control agent for tradescantia, it wouldn’t be a disaster.
There have now been four agents – three insects and a fungus – introduced to control tradescantia. When I was last in Auckland, I was excited to see leaves which had been eaten by one of the beetles. I realised then that I had never before noticed leaves of tradescantia with insect damage. I’m still waiting to see whether it’s going to be enough to shift the balance in restoration sites so that native seedlings can get through a patch of tradescantia. That will take time to know.
In contrast, one of New Zealand’s biggest weed problems is the spread of wilding conifers, particularly species like lodgepole pine. Even from the common name, you can probably guess the problem with biological control for that one. The scientific names confirm it. Lodgepole pine is Pinus contorta. Our most important forestry tree is Pinus radiata. Our second most important is Douglas fir, which belongs to the same family. I know that there have been discussions about biological control for wilding conifers, because the problem is so severe. But any mistakes could be catastrophic. (I realise that some people hate pine trees, so of course this is a matter of value, not a matter of fact. There is also the problem that both radiata pine and Douglas fir are themselves invasive in some circumstances, but I’ll write more about that another time.)
My work was biosecurity and not biological control, but some of the same principles applied. When I looked at insect pests and diseases overseas, I looked at the plants that they ate or infected, then I looked at what those plants were related to. Of course, some of the pests and diseases were like goats and affected many different, unrelated plants. Predicting what they might do in New Zealand was particularly tricky. Usually, that was a matter of looking at where they were a problem overseas, and looking at how similar the environment was to New Zealand. An insect which thrives in England, south-eastern Australia or Uruguay is far more likely to be a problem than an insect from Malaysia or Fiji, for example.
Sometimes, our best prediction was that the impacts would be unpredictable. There’s a type of bacteria which can cause serious disease in plants and has spread to new areas in the last couple of decades. It goes by many different names, because in every plant species it infects, the disease looks different. Until they were able to use modern genetic methods to study it, scientists didn’t know that they were looking at the effects of the same bacteria in different plants. So, in grapevines the disease is known as Pierce’s disease, in olives, the disease is olive quick decline syndrome, in coffee it causes coffee leaf scorch and in peaches it causes phony peach disease (so-called because the plants appear healthy but produce only a few poor quality fruit).
None of these plants are related to each other. Nor do they necessarily grow in the same environments. Not only that, but when the bacteria arrived in Europe in 2013, it began to affect a whole new range of plants. It seemed as if every month or so, I would hear about it being recorded on a new plant species. What was going on?
Just as mosquitoes can spread diseases from one human to another, some types of insect can spread diseases from plant to plant. These bacteria were being spread by certain kinds of sap-sucking insect. What mattered was the types of sap-sucking insect present in an area, and the plants they were feeding on. Should the bacteria ever reach New Zealand – and I hope that they don’t – then which plants get affected will depend on which of the insects present here are able to spread it, and what they are feeding on. In theory, we could test the insects we have here, but that is harder than it sounds. Either we’d have to bring these very damaging bacteria to New Zealand and keep them contained, or we’d have to send a whole lot of different insects to the far side of the world and establish colonies, again keeping them contained, and test over there. In practice, this is unlikely to happen. So, all we can really predict is that it would be unpredictable.
Among the pests and diseases which I looked at in my years of biosecurity, there’s one which stands out. It’s another example of a predictably unpredictable species, this one a type of fungus known as a rust fungus. Its story is fascinating to a botanist, because it confines itself to infecting a single family of plants. But it’s also heartbreaking, because the damage it does can be severe, and it is now threatening some plants in Australia and New Zealand with extinction.
The fungus is myrtle rust, and I’m going to write about it in my next couple of articles. The islands of Hawai’i also play an important part in this story, and that is also the island group I’m going to write about next.
Good article. You might have mentioned that some botanists…and others….have corrupted Linnaeus method by naming plants not after features of the plant, but after their friends.