The sight of clear water flowing under the deep shade of a forest canopy is a balm. It’s as if my fevered spirit is one of the stones, cooled by the stream’s caress. I know this is how a stream is supposed to run – not through open fields but through trees and shrubs and ferns and mosses. It looks right. It feels right.
But it isn’t always possible to tell if a stream is healthy just by looking at it. Many of Wellington’s streams look good when they are flowing though forested gullies, but this doesn’t mean they are clean. I saw the horrifying evidence of this when I walked in one of the gullies near the Ngā Ūranga gorge, and saw where a sewage pipe regularly overflowed into the stream.
When we study the health of a stream, one of the things we do is test for certain microbes. The one we hear about most often is usually known as E. coli. It’s much maligned for its association with sewage, but there’s so much more to this humble microbe. In fact, we have harnessed it in quite extraordinary ways. It has helped us advance our scientific knowledge and has contributed to saving many lives.
The 19th century saw huge advances in our understanding of microbes. This was the era, for example, where western medical doctors finally learned that it was a good idea to wash their hands. There’s far more to that story, of course, because there were others who knew before them, but that’s a story for another time. Another important step in our understanding of microbes was the discovery of how to separate different types of microbes and grow pure cultures, which contained only a single type of microbe. The development of pure cultures allowed scientists to categorise microbes in a way they’d never been able to before. They began culturing samples from all kinds of places, separating out the different types and giving them names.
One of these scientists was Theodor Escherich, who discovered a type of bacteria in the human intestine (it was later found in the intestines of other warm-blooded animals as well). He named it Bacterium coli, since it was found in the colon, but the first part of the name would later be changed to honour its discoverer, and so it become Escherichia coli. This name is such a mouthful that it’s always spoken of as E. coli.
It turned out that these bacteria aren’t particularly abundant or important in our intestines, but they have one outstanding feature – they are exceptionally easy to grow in a laboratory. This is what makes E. coli such a good indicator of sewage contamination. If you take a sample of water from a stream, grow the bacteria from the water and don’t find E. coli, then you can be sure it’s not there. However, if it is, that’s a clear sign that the contents of an animal’s intestine (i.e. poo) has found its way into the water. And that means it’s likely other microbes, which are more dangerous but less easy to grow in a laboratory, are present as well.
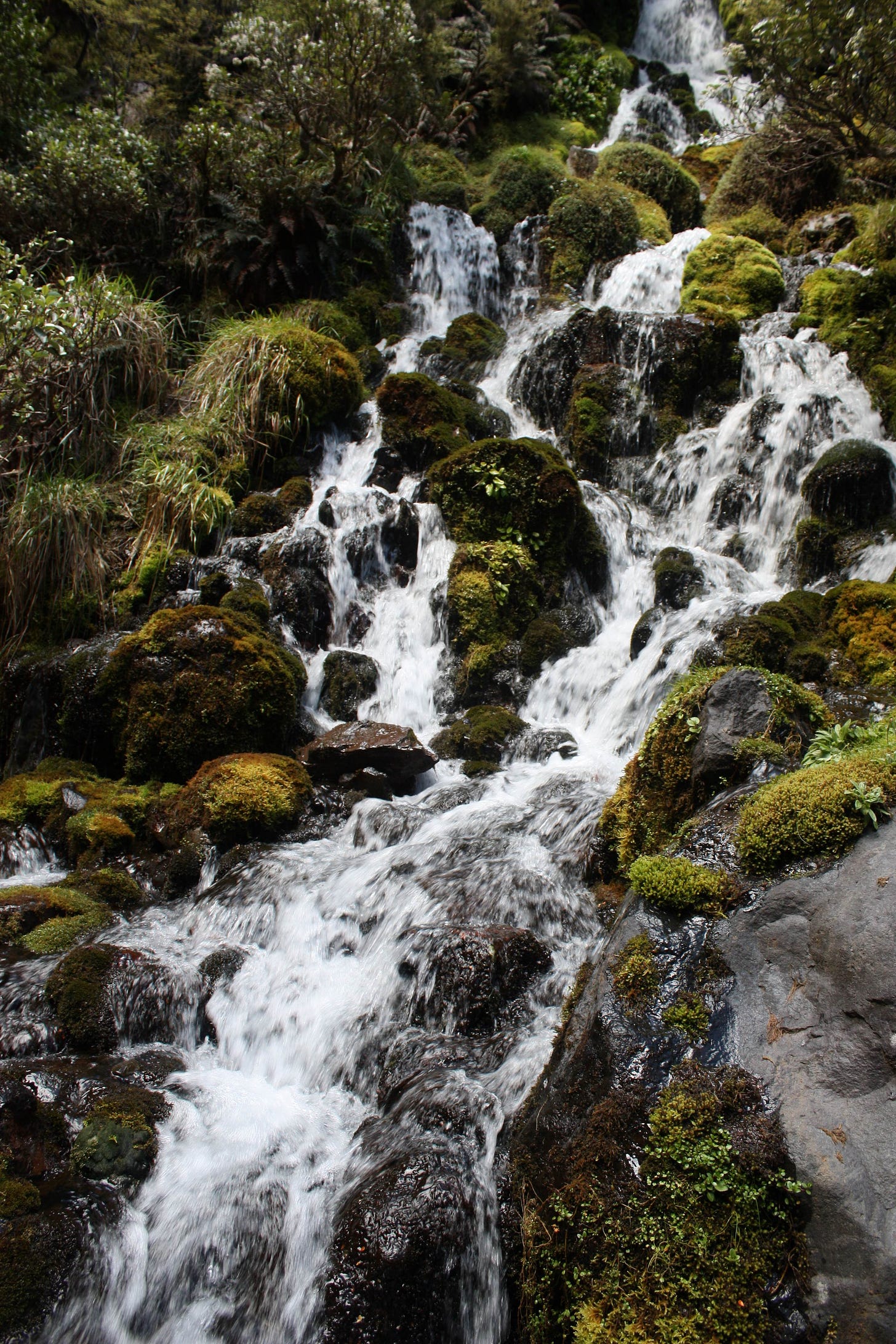
When scientists were trying to better understand how cells worked, they looked around for something easy to culture, fast growing and available. E. coli ticked the right boxes. It was also safe to work with, because although there are strains which can cause serious diseases, there are plenty of strains which are harmless. Because bacteria have simpler cells and a simpler way of reproducing than larger life forms such as fungi and humans, they are useful for studying genetics. Much of what we know about the nature of genes was originally studied using E. coli.
Like other bacteria, E. coli shares genetic information on small rings of DNA known as plasmids. In 1973, work on the plasmids of E. coli led to a powerful but terrifying breakthrough. The previous year, Stanley Cohen, who studied plasmids in E. coli, travelled to Hawai’i for a conference. Also attending the conference was Herbert Boyer, who was working on ways of cutting DNA molecules using enzymes. Boyer’s laboratory had discovered a new way of cutting the two-stranded molecule of DNA which left one of the two strands longer than the other (I’ve written previously about the structure of DNA if you want more background on this). Because of the way that DNA strands react, if one of these uneven molecules met another uneven molecule with the right piece of code, they would latch on to each other, forming a new piece of DNA. Already, another scientist, Paul Berg, had used the technology to create DNA molecules which combined the genes of two different species.
The two scientists decided to collaborate. They began chopping up plasmids containing a gene for resistance to the antibiotic streptomycin, joining them up with other pieces of DNA, and then putting them into E. coli cells. Sure enough, the E. coli cells could grow in the presence of streptomycin. They then added genes for resistance to antibiotics, some of which had originally come from different species of bacteria. This resulted in E. coli which could resist the other antibiotics as well. They had succeeded in creating the first transgenic organisms, that is, organisms which incorporated the DNA of more than one species.
Outside of a laboratory, creating new types of antibiotic resistant bacteria is the last thing we want to do. But for the purposes of research on genetic modification, antibiotic resistance proved crucial. Scientists were not necessarily able to tell whether they had succeeded in adding a new gene to a cell’s DNA, even with a powerful microscope. So, they began bundling genes for antibiotic resistance together with the genes they were studying and attempting to introduce into new species. Then, they would include antibiotics when they were culturing the resulting cells, meaning that only the modified cells would survive.
The scientists doing this work were not blind to the risks of chopping and changing an organism’s genes in this way. Paul Berg, in particular, was very conscious of the potential for harm. He held back from some of his planned experiments because of concerns about safety, and in 1975 organised a conference which would set up guidelines on genetic modification research. I’ll write more about this conference, some of Berg’s research and some of the risks another time. It’s an interesting case where scientists developing a new technology worked together to try and ensure they didn’t create a disaster, before any problems had developed. For now, though, I want to mention one of the most important safety measures – containment. If a genetically modified organism is contained rather than being released into the environment, many problems can be avoided.
Around the time of the conference, researchers were recognising the potential of genetic modification for manufacturing protein molecules. Life is built on the translation of DNA to molecules known as amino acids, which are the building blocks of proteins. Could we genetically modify cells such as those of the microbe E. coli to manufacture medically useful proteins, such as insulin, which we couldn’t manufacture any other way? If we could, there would be no need to release the modified microbe into the environment, we could simply separate out the molecule we needed and leave the modified microbe behind. It was an approach with much lower risks than releasing a genetically modified organism.
Doctors have recognised the symptoms of type 1 diabetes for hundreds, if not thousands, of years. Until the discovery and commercial production of insulin in the early 1920s, the condition was a death sentence. Insulin was a life-giving miracle, but a lot of it was needed. One of the first people treated with insulin went on to live for another 58 years, receiving more than 42,000 insulin injections. And there was only one way to produce insulin – extracting it from the pancreases of animals such as pigs, cows or horses.
By the late 1970s, scientists were good at chopping up pieces of DNA and splicing them together, but there was a problem with the gene for insulin. A gene is simply a piece of DNA where the individual components, known as bases, are in a particular order. But scientists didn’t know what the order was. Because they didn’t know what the order was, they couldn’t find it and make a copy from a human cell. But they did know the structure of the insulin protein, so they worked backwards – looking at the individual amino acids of the insulin protein and then working out the order of the bases from that. Once they had the order of the bases, they were able to join chopped up fragments of DNA to make the gene for insulin.
Then they took their gene, inserted it into a plasmid and then into E. coli. And it worked – the E. coli produced insulin. It was of a higher quality than insulin extracted from animal pancreases too, and didn’t cause allergic reactions as animal insulin sometimes did. Although it took a few more years before they were able to produce enough for it to be commercially useful, they had made a stunning and life-saving breakthrough. Since 1982, most the world’s insulin supply is made either by E. coli or by a type of yeast which has been genetically modified using a similar technique. There are also many other therapeutic compounds produced by E. coli.
Well, that’s what most of the histories say. In fact, there’s a detail in the history of insulin production which is often left out, but is worth noting. The scientists doing the original research, including Boyer and others working for the biotechnology company he set up, were worried about safety. Was it really a good idea to have bacteria which could survive in the human gut making a protein like insulin which could be dangerous in the wrong amounts and in the wrong place? What if it escaped? So, they designed their gene not to make the whole insulin molecule, but to make it in two separate parts. Once they had purified two parts, they could be combined to make active insulin.
There is much to consider in the safety of genetically modified organisms. Not every use of the technology is as clearly beneficial as producing insulin. Not every scientist working on it is as ethical and conscientious as Berg and Boyer. But the safe and efficient production of insulin is a reminder of the potential of new technologies. We can use them to do great things. Whether or not that happens depends on the decisions of scientists, regulators, companies. Those decisions depend, to some extent, on what we as a society are saying. So I hope that this article sparks some conversations.
This week I’m at a conference on plant conservation in Whangārei, so next Sunday’s article will be about plants, but I’ll be back to genetic modification in a couple of weeks.
Fascinating and useful background for people like me who have never studied genetics..
Really interesting! I had no idea of that connection between insulin and E. coli