There wasn’t much I enjoyed about doing my Masters degree, but I do remember that the liquid nitrogen was fun. I was supposed to be studying a group of viruses which infected grapevines, using what were, in the early 1990s, new and interesting techniques. First, I was supposed to extract the genetic material of the virus from infected grapevines. I don’t exactly remember what I was supposed to do beyond that, because I basically failed at the first hurdle. Grapevines were already notoriously difficult to extract genetic material from. There was a published method, but for some reason various people had made “improvements” to this method, and that was what I was told to work with. It took some time for me, and my supervisors, to recognise that the supposed improvements were one reason nothing worked for me. Only when I reverted to the published protocol did I begin to make progress.
In the meantime, I used a lot of liquid nitrogen. The viruses I was studying were most abundant in a thin layer of tissue found just below the bark of the vines’ branches. I had to take a length of stem, peel away the bark, then carefully scrape off that tissue layer and grind it as finely as possible, to use it for extraction. To grind the scrapings, I would drop them into a mortar containing a small amount of liquid nitrogen, which would freeze them so cold that they could be crushed to a powder. This wasn’t easy – liquid nitrogen is extremely cold, boiling away at -196oC (that’s -381oF for American readers). It has to be stored in insulating canisters which could withstand high pressures from within, because as it warms it expands rapidly. I had to wear safety goggles and heavily insulated gloves to pour a small amount from the huge canister into my mortar. As I ground the scrapings, the nitrogen would be boiling away, clouds of it swirling from the mortar and pouring over the lab bench as if I was playing one of the witches in Macbeth.
I haven’t brought up this memory to explain about liquid nitrogen though. It’s relevant to me because when I picture that laboratory, I see two squat machines sitting on one of the benches. Those machines take me to the remarkable tools scientists use to understand, and manipulate, genetic material. Understanding how these tools work helps us understand what scientists are doing when they modify genes.
The machines I remember from the laboratory were early models of the machines used for a type of analysis known as PCR, not far advanced from the one pictured below. PCR may sound familiar, because PCR tests were widely used during the COVID-19 pandemic – tens of thousands of tests were processed in New Zealand every day from mid-2021 until mid-2022, and there are still thousands being done every week. Those kinds of numbers were incomprehensible in the early 1990s. I don’t remember the details, but I do remember that PCR was a complicated, time-consuming process.
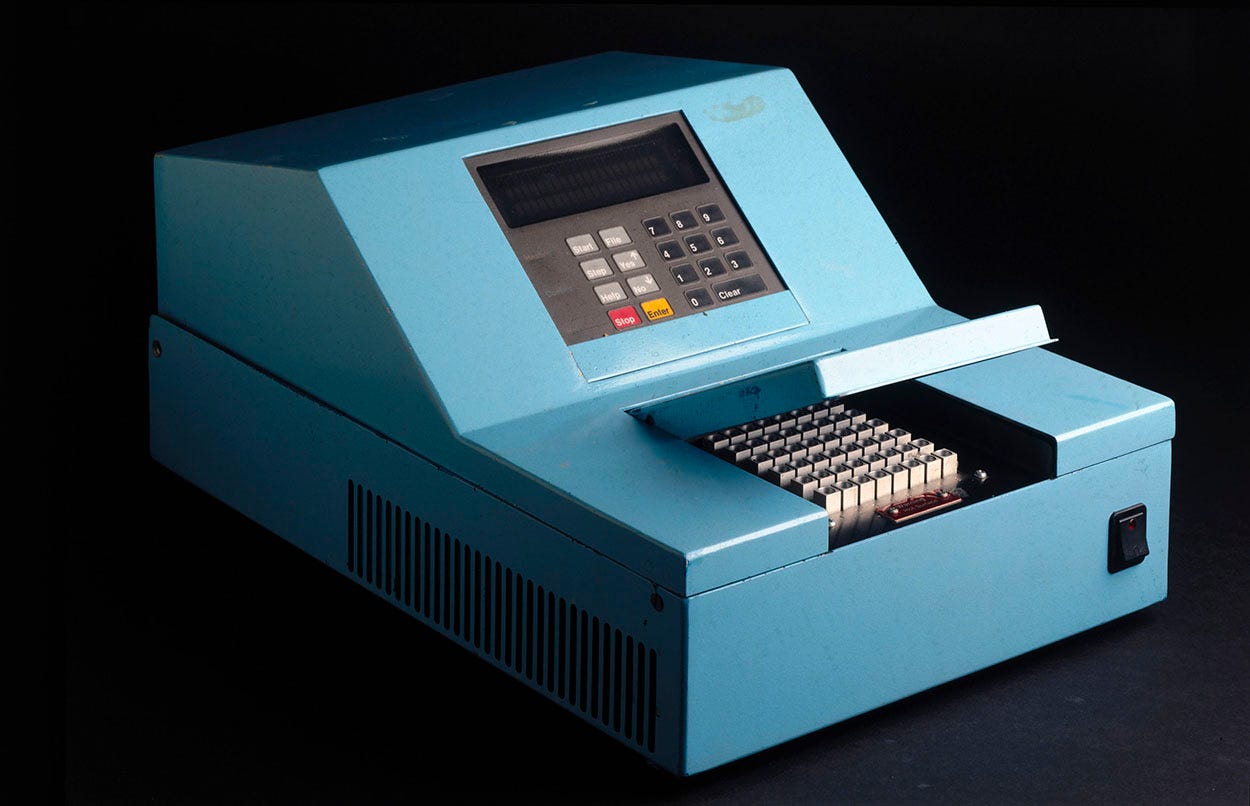
The PCR machines themselves weren’t anything remarkable. In effect, they were, and still are, very precise ovens with a timer. They rapidly heat and cool samples to the required temperatures and lengths of time, over and over again. The interesting tool is something added to the samples, an enzyme isolated in 1976 from bacteria discovered growing in a hot spring in Yellowstone National Park. This discovery is worth highlighting, because it’s a reminder that world-changing breakthroughs are often built on apparently esoteric science with no obvious use.
Some of you will be old enough to remember the advertisements for laundry detergent with hungry enzymes, little green critters which sucked the stains from clothing (here’s a classic one, for the sake of nostalgia). Enzymes aren’t little green critters, of course. They are molecules, usually proteins, which control the chemical reactions inside living cells.
Because enzymes are molecules found in living things, they usually operate at temperatures favourable to life. If they are heated too much, they are destroyed, or rather irreversibly changed. Think of what happens when you boil an egg – the change you see is a change in the structure of their proteins. There’s no way to unboil an egg.
But the bacteria in that thermal spring were adapted to high temperatures, so their enzymes could survive heating. One of those enzymes made copies of genetic material (or DNA). Why was it so important? I’ll have to take a step back to explain.
DNA molecules are massive. Ours have 3 billion individual units, known as base pairs. There are four different kinds of these base pairs. It’s the specific order, or sequence, of those base pairs which makes up our genetic code (there’s more detail on this in an earlier article, so I won’t repeat it here). Unless we are an identical twin, each of us has a unique sequence of base pairs on our DNA, that is, a unique code. But, because our DNA is a combination of DNA from our parents, the vast majority of our code is identical to the code carried by each parent. If we have siblings, then they will also have most of their code identical to their parents, and therefore sections of code identical to ours. If we work backwards, to our grandparents and great-grandparents, or sideways to our cousins, there are slightly more differences in code, but it’s still very similar.
In fact, there is vastly more code which we have in common with all humans than there is code which is different. We share 99.9% of our DNA with all other humans. We also share most of our code with chimpanzees, the exact percentage quoted differs because there are different ways of measuring it, but it’s around 98-99%. In fact, there’s a lot of code which all living things have in common. It isn’t particularly meaningful to quote percentages, because there are different ways of describing and measuring similarity. But there are sections of code, or sequences, which are shared by all humans, but not other apes; sequences shared by all mammals, but not birds or insects; and sequences shared by all animals, but not plants.
I’ve written before about the way learning to read these sequences changed our understanding of the relationships between living things. But the part of the story I didn’t tell is the role of the enzyme from the Yellowstone bacteria.
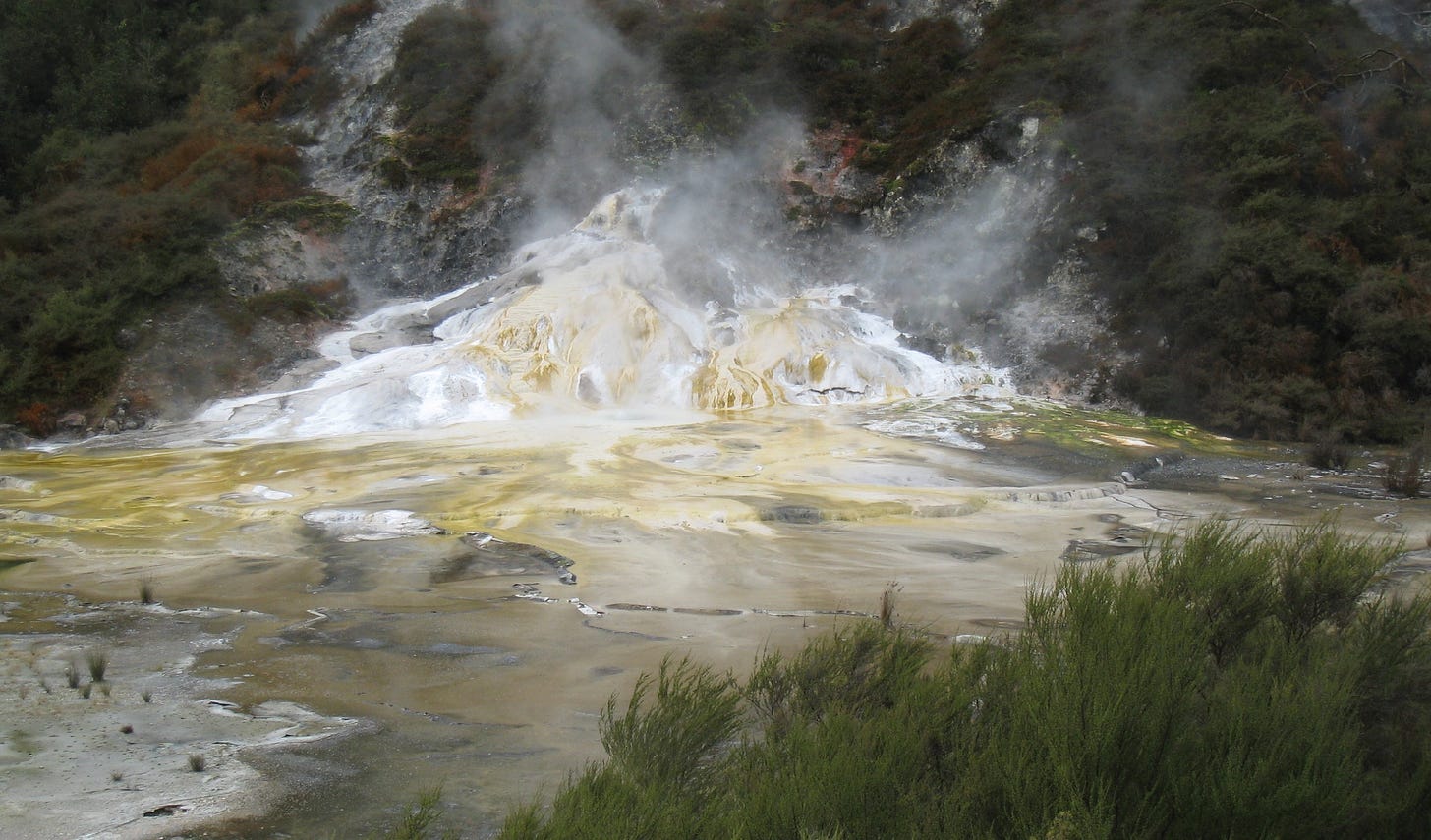
When I was grinding up my grapevine scrapings, I would end up with around a tablespoon of powdered tissue. I would then go through a chemical extraction process, and end up with a couple of millilitres of liquid in a tiny plastic tube. Even then, the amount of genetic material in the sample was tiny and difficult to detect. It would have been much easier if I had some way of making lots of copies of that genetic material.
This is where the enzyme comes in. It’s a genetic photocopier, able to make copies of DNA. To do this, though, the two individual strands of DNA which are twisted together need to be separated. This is done by heating the DNA to 94oC (201oF). The temperature then needs to be lowered so that small pieces of DNA known as primers can attach to the strands. Primers indicate where the DNA copying should start and end. Then, the temperature is raised again, although not so much, for the enzyme to copy the DNA. The process is repeated, again and again. The first temperature cycle doubles the number of DNA strands, then the next cycle doubles those, and by the time there have been 25-35 cycles, there are millions or even billions of copies. Here’s a link to a video explaining the process.
Before the discovery of the enzyme in the Yellowstone bacteria, scientists used similar enzymes from the laboratory workhorse, E. coli, a type of bacteria found in the human gut. E. coli is a tough little microbe, but it can’t survive 94oC, and nor can its enzymes. As a result, when scientists were originally trying to multiply DNA, they had to add new enzymes after each heating step. Once they began using the enzyme from the Yellowstone bacteria, they could add it at the start and leave the PCR machine to run through as many temperature cycles as they needed.
The enzyme first found in the Yellowstone bacteria, and its use in PCR tests, has revolutionised the diagnosis of disease and forensic science. It is also an essential research tool. But there are other enzymes which can manipulate DNA, and they may turn out to have an even greater impact on our lives.
Enzymes can do more than copy DNA – they can also cut it. In the late 1960s, scientists working with – surprise, surprise – E. coli, discovered an enzyme which would cut DNA if it recognised a particular sequence of base pairs on that DNA. It didn’t, as I expected when I first read about it, cut at the point where it recognised the sequence. It cut the DNA at a random location. But this does make sense – E. coli was using the enzyme to attack a virus which infected it. If the enzyme recognised a particular sequence characteristic of the virus, it chopped up the DNA and destroyed the virus. In effect, it was like a bacterial immune system.
Scientists soon discovered more of these enzymes, some of which would cut DNA at specific rather than random locations. Once again, an apparently arcane endeavour was beginning to look as if it might have a practical application. Initially, scientists used these enzymes to help them map genes. But, as I wrote a couple of weeks ago, the discovery of an enzyme which made an uneven cut, again in E. coli, raised another prospect. If you’ve ever played with lego, you’ve probably seen how overlapping ends can be used to join lengths together. Could DNA fragments be joined together in a similar way?
As it happens, enzymes for joining DNA together were also discovered around this time. If you haven’t already guessed, they were first discovered in E. coli. However, it wasn’t long before it was discovered that the enzymes were everywhere. We all have them, and they are used by our cells when repairing and reproducing our DNA.
The combination of cutting enzymes, copying enzymes and joining enzymes gave scientists a powerful set of tools to multiply DNA, chop it up and combine it in new ways, creating entirely new DNA molecules. Scientists began these experiments in the early 1970s, and within a decade, had created the genetically modified E. coli which now produces much of the world’s insulin supply.
Today, I’m not going to look further at how scientists used this technology, although I will return to that topic, because any discussion of gene technologies must consider this point. In my view, there is a world of difference between producing a safer, more reliable supply of insulin, and making golf course grasses resistant to herbicide in order to produce a better green.
Instead, I want to look at one more enzyme, or group of enzymes, known as Cas, which are crucial to the discussion of genetic technologies. Technically, they are chopping enzymes, but there’s a difference in the way that they are directed to where they chop DNA. Their discovery has been every bit as revolutionary as the discovery of the enzyme in the Yellowstone bacteria.
For once, E. coli wasn’t central to the discovery of the Cas enzymes. Instead, they were first discovered in bacteria associated with a wide range of human illnesses, including sore throats and rheumatic fever. However, like the chopping enzymes first discovered in E. coli, the Cas enzymes were part of the bacterial immune system, chopping up the genetic material of viruses.
Cas enzymes are precisely piloted by small pieces of genetic material known as RNA. It’s very similar to DNA, but is usually single-stranded. In bacteria, this RNA was created using a specific segment of DNA. Although I have already used more than my quota of acronyms today, I need to mention this one, because it comes up a lot. The segment of DNA is known as CRISPR, and it’s this acronym which is often used when talking about the Cas enzymes. In fact, it’s the enzymes and the guide RNA which are important, but CRISPR is catchier, and the name has stuck.
In 2012, Jennifer Doudna, Emmanuelle Charpentier and colleagues published a paper explaining the workings of CRISPR and Cas, noting that it could be used for a “programmable” system to edit genes. Based on what I’ve read about the impact of their discovery, it was like moving from a typewriter to a computer for writing and, more to the point, editing.
The CRISPR system has allowed scientists to do much more with genes than they ever could using the earlier methods. As I read about it, I have the same kind of feeling I have about artificial intelligence – that technology is racing away at a pace far beyond my comprehension. Sometimes, I think about the English textile workers in the early years of the 19th century, who smashed mill machinery as a form of protest. They gave a new word to the lexicon – Luddite – which has come to mean people who struggle with new technology.
But a closer look at the Luddites reveals a more nuanced image. Luddites weren’t opposed to the use of machinery. They were protesting low wages and the use of the machinery in a “fraudulent and decietful manner” to get around established labour practices. If I think about what troubles me in genetic modification, and in artificial intelligence for that matter, then I realise that much of my concern is about how the technology is used – and who decides how it is used. My other concern is about the risks and, again, who makes those decisions.
So, that’s where I need to go next. How are genetic technologies like CRISPR being used? What are the risks? And how do we make good decisions about how to manage them?
As well as "making golf course grasses resistant to herbicide in order to produce a better green" it increases sales of said herbicide. Not a good use of gene technology, in my opinion.
This was a very thorough overview. I was especially amazed by the sheer number of footnotes to your prior writings. I still have a lot of catching up to do. For those interested in the topic I thought the Walter Isaacson biography of Jennifer Doudna (The Code Breaker) to be excellent. I have read many of his biographies and always walk away having learned something and his shared insights into what the outliers amongst us can deliver to the world. I find his approach to biography to be striking and found Doudna to be an outlier amongst people who have changed the world. She does not seem to be attracted to fame, fortune, and narcissism as seems to be a common downfall. I would imagine if people read his profiles of Steve Jobs or Elon Musk, they might reach for the vomit bag as these are simply very broken humans.