Welcome to The Turnstone. Here, I help people understand important issues such as Covid-19, climate change and conservation. I send my articles out every Sunday - if you’d like them emailed to you directly, you can sign up to my mailing list.
In the northern Pacific Ocean, between the coast of California and the island chain of Hawai’i, is a slowly rotating area of plastic waste known as the Great Pacific Garbage Patch. It’s not, as I imagined before I began researching this article, an island of plastic floating on the surface. The plastic waste in the Great Pacific Garbage Patch extends from the surface of the ocean to the sea floor. Almost half of it is made up of discarded fishing nets, and around 8% is made up of microplastics. Scientists estimate that there are around 1.8 trillion pieces of plastic scattered across an area twice the size of New South Wales.
But the eastern Pacific isn’t alone in having a giant patch of plastic waste. There’s at least one more in the Pacific. The Indian Ocean has one as well. The Atlantic Ocean has two, one in the north and one in the south.
These accumulations of plastic debris are a visible sign of something invisible: currents in the ocean. Specifically, such patches form where the ocean currents form something called a gyre, which is an area of rotating ocean current. Gyres are formed from a complex interaction of the prevailing wind and the rotation of the earth, and to understand them, it helps to take a step back and look at the discovery of something called Ekman transport.
I have to admit, part of the reason I think it’s useful to go back to the story of how Ekman transport was discovered is because it’s linked to one of the greatest figures in polar exploration, Fridtjof Nansen. Not only was Nansen an explorer, he was also a scientist, diplomat and humanitarian (among other things, he was the world’s first High Commissioner for Refugees). In 1898, as part of his expedition to the North Pole, Nansen deliberately got his ship, the Fram, frozen into the sea ice. His theory – the ocean current would take the Fram to the North Pole. It didn’t, but Nansen didn’t waste the time he spent waiting. Among his many careful observations was one that sea ice did not move in the same direction as the wind, but at an angle of 20-40 degrees to the right of the direction the wind blew. He believed that this was the result of the rotation of the earth.
A Swedish oceanographer, V. Walfrid Ekman, investigated Nansen’s observation and turned it into a mathematical model. What he found was that as the water got deeper, the speed of water movement slowed, as some of the energy from the wind was lost. But there was something else – as the depth of the water increased, the angle between the direction of the wind and the direction of water movement increased as well. The combination of reducing energy and increasing angle meant that, on average, water currents move at around 90 degrees to the wind direction. In the Northern Hemisphere, this movement is to the right, as Nansen observed. In the Southern Hemisphere, it’s to 90 degrees to the left. This water movement is known as Ekman transport, since Ekman first described it in detail.
If this all sounds confusing – I know it had my brain in knots at first – it’s explained very well in this video.
Ekman transport results in all sorts of interesting things happening in the ocean, not just gyres. Where winds blow parallel to coastlines, they can cause currents which either flow towards the shore or away from the shore, depending on the direction of the wind and which hemisphere it’s in. When Ekman transport carries water towards the shore, the water has to go somewhere – it can’t just pile up forever. What happens is that it creates a downward current, called a downwelling. And when the wind causes the current to flow in the other direction, away from the coast, there is an upward current from the ocean’s depths, called an upwelling.
Upwellings are important because they carry cool, nutrient-laden water from the floor of the ocean to the surface. Areas with upwellings are highly productive. For example, the upwelling off the coast of Peru – part of the Humboldt Current – produces about 10% of the world’s fish catch from an area which is less than 0.1% of the world’s ocean. Upwellings are important for biodiversity as well. For example, the Benguela Upwelling System, off South Africa’s west coast, supports particularly diverse populations of marine life and seabirds.
Currents are important for other reasons too. Western Europe is, on average, 10oC warmer than other places at equivalent latitudes. The reason, at least in part, is a current commonly known as the Gulf Stream. This current takes warm water from the area off the coast of Florida and carries it to the northern Atlantic. Ocean currents, too, play a role in the El Niño and La Niña weather patterns that have such an influence on the climates of Australia and New Zealand.
The ocean, then, is in constant motion. Water seldom stays in one place for long – it wells up or sinks down, circulates in giant spirals, pours through narrow gaps and rebounds off continents. Although we can’t see this motion directly, it still affects us.
But something is happening to ocean currents. As the world’s climate changes, driven by carbon emissions into the atmosphere, the ocean currents are changing too. Some currents are slowing down. Others, scientists discovered recently, are getting faster.
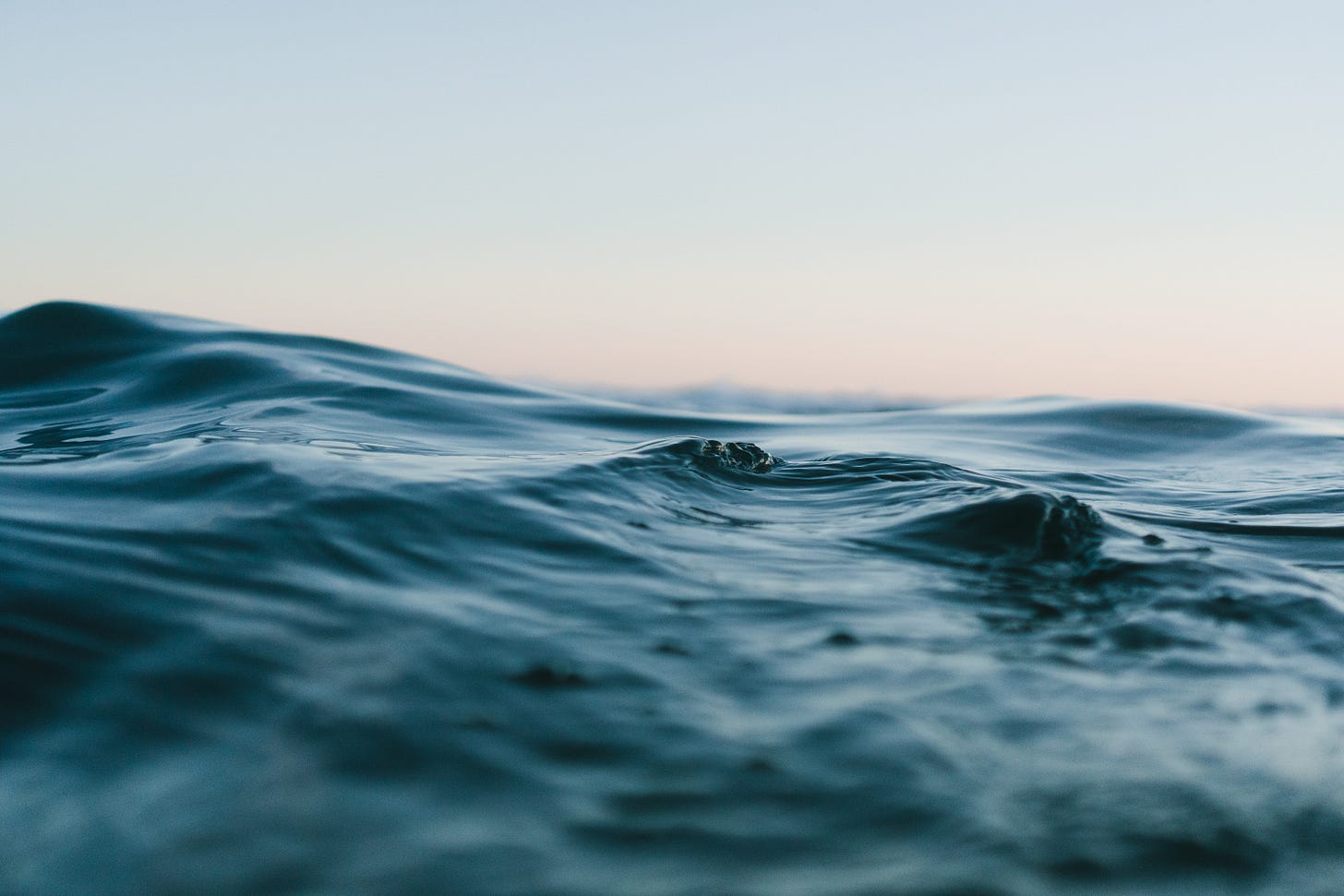
The ocean current which has had the most attention is the Gulf Stream, which is actually part of a much bigger system known as the meridional overturning circulation, although it’s also informally known as the global conveyer belt, which is less of a mouthful. This is a huge ocean current, carrying water around the entire Atlantic, around Antarctica, up into the Indian Ocean and in a big loop around Australia and New Zealand. Some of the water travels at the ocean’s surface, while other water travels at depth. There’s a great video describing it here, although it’s focused more on the part of the current affecting the Atlantic.
For the last 5000 years, the global conveyer belt has been relatively stable. But, over the last few decades, there’s some evidence it has been slowing down and perhaps changing its course. The reason has to do with the melting of Greenland’s ice sheet. As the ice sheet melts, it pours fresh water into the northern part of the Atlantic Ocean, in the area where water which has been travelling north starts to sink. That dilutes the sea water, making it less salty. But why does that make a difference? The saltier water is, the heavier it is – less salty water is lighter so it doesn’t sink as fast, and that slows down the current.
What does this mean? At this stage, it’s not clear. Scientists have proposed some scenarios where the global conveyer belt collapses, which would have far-reaching impacts on the global climate. But even if that doesn’t happen, a slowing of this current could cool western Europe and raise sea levels by an extra 10 cm on the east coast of North America.
But that’s not the only thing happening to ocean currents because of climate change. In contrast to the global conveyer belt, other ocean currents are actually speeding up. This is a very recent finding, first reported just two years ago. At first, scientists assumed that this increase was the result of changes in the wind. However, further work has shown that it’s actually the warming ocean temperatures which have caused this increase in speed.
I’ll take a deep breath here and say that this phenomenon has taken me even longer to understand than the slowing of the global conveyer belt. But I think I’ve got it.
The ocean is not at a uniform temperature. Because most of the heat which warms the ocean comes from the sun (apart from a few undersea volcanoes), the ocean surface is the area which warms up the most. In most parts of the ocean, apart from the poles, there is a temperature gradient, where it gets cooler the deeper you go (the fact that cooler water is more dense also contributes to this gradient). Warm water at the ocean’s surface doesn’t mix easily with the cooler water at depth – not unless there’s a current like the global conveyer belt driving it.
As the atmosphere warms and transfers more warmth to the ocean, the gradient increases and the layers of water are even less inclined to mix. In effect, the water at the surface has less vertical space to move in. And when flowing water has less space, the speed of the water flow increases. This is called the Venturi effect, and you’ll have observed it if you’ve put your thumb over the end of a garden hose to make the water spray out further.
This is still a new finding, so it’s not clear what it will mean. Placed alongside the increased frequency of extreme weather events we are experiencing now and the sea level rises that future generations are facing, subtle changes in ocean currents might not seem like such a serious problem. But they are another illustration of the complexity of the world we live in, and a reminder that we can’t wait until every question is answered before we take action.
The Turnstone is free, but if you would like to support my work with a monthly or annual subscription, click the “Subscribe now” button below for options.
If you would like to support The Turnstone with a one-off contribution, click the “Buy me a coffee” button below.
Really interesting - the more you write, the more I find I don't know, especially about the ocean. Love the analogy about the hose for the Venturi effect - that painted a very clear picture. I think one of our biggest faults as humans is our tendency to compartmentalize, when really what we need to understand is how very interconnected everything is.
so interesting! thank you melanie!