Driven to destruction
The strange science and troubling potential of gene drives (10 minute read)
Before I start, I did a quick live video chat with Stephanie Losi of Risk Musings, in preparation for the course I’m teaching about demystifying risk to health, the environment and society.
You can check out the video below:
What I wish people understood about risk
In preparation for my course on Demystifying Risk, I chatted with Stephanie Losi from Risk Musings. Stephanie’s going to join me as a guest lecturer for the course.
The Bloodsuckers exhibition at Auckland Museum a few weeks ago wasn’t the first exhibition I’d seen on parasites. Many years ago, I saw another exhibition. It may also have been at the Auckland Museum, but it’s so long ago I can’t remember. I also don’t remember what the exhibition was about. I remember one thing from it though – a truly terrifying fact about mites.
These tiny creatures are not a kind of insect but are cousins of the spider. They are an extraordinarily diverse group, but because they are tiny, they don’t often get much attention. Gardeners may have encountered the red spider mite. Beekeepers will certainly know of the varroa mite. But even though we all have them, almost nobody knows about the eyelash mite.
I don’t think I’m particularly squeamish, but I was horrified to hear about eyelash mites, which, as their name suggests, live on our eyelashes. Strictly speaking, they probably aren’t parasites, since parasites are by definition harmful to their host. Eyelash mites are more correctly considered commensal organisms, which means that they benefit from us without causing us harm, unless they get out of control, which doesn’t often happen. They might be harmless, but I still found the mere thought of them disturbing. After a while, though, I got used to the idea that we all carry these little companions.
After the eyelash mite, I didn’t think I would ever be surprised about a parasite again. But they still have ways of surprising me. Wolbachia, which I wrote about last week, was certainly something I didn’t expect.
Then I began looking into another approach to mosquito control, known as a gene drive. And I realised that I needed to revise even my basic idea of what could be a parasite. I’ve heard about parasites from a wide range of animal groups. I know that there are many kinds of parasitic plants, from those which are entirely parasitic to those which are only slightly parasitic. I’m familiar with many microbial parasites, and even some which sit on the boundary between living and non-living, like viruses (there are also viroids and prions, which I’ll write about one day).
However, a gene drive involves a completely different kind of parasite, something that we wouldn’t expect to be parasitic at all – a gene, that is, a mere piece of DNA.
What is it that makes a gene parasitic?
The basic premise of evolution is that a living thing with beneficial traits is able to survive and reproduce better than another living thing with traits which aren’t so beneficial. Charles Darwin had no idea about how those traits were passed on, only that it happened. When scientists learned about genes, the picture became clearer. It wasn’t so much the beneficial traits which were passed on, but beneficial genes. For more information, I’ve linked to a video which explains more about the process.
In the 1920s, a Russian scientist studying obscure fruit flies1 from around Moscow found something odd. A couple of female flies laid eggs which developed into predominantly female offspring, with very few males. Aha, you might be thinking if you read last week’s article. Could the flies have been infected by Wolbachia?
But this was not Wolbachia. The flies who produced predominantly female offspring weren’t wild flies, but ones which had been inbred. Inbreeding tends to show up strange, hidden mutations – so something odd was happening with the genes. By making a series of different crosses between normal flies and flies from the predominantly female group, the scientist managed to pin down exactly what was going on.
In every living thing, genes are carried on structures called chromosomes. Some of these chromosomes are designated as sex chromosomes, because they are different for different sexes. Fruit flies have the same basic sex chromosomes as humans, an X and a Y2. Females usually have two X chromosomes and males one X and one Y. When parents pass on their genes to their offspring, they pass on half of their chromosomes, which means the female parent is guaranteed to pass on an X chromosome, but the male passes on either an X or a Y, resulting in either female or male offspring.
One similarity between humans and fruit flies is that the Y chromosome is much smaller than the X and doesn’t carry as many genes. So a female, with two X chromosomes, gets two copies of all of the genes on the X chromosome. But a male gets only one copy of the genes on the X chromosome, along with whatever is carried on the Y. If there happens to be a faulty gene on one of the X chromosomes, a female is usually okay, because she has two copies of the X chromosome, and the chances are that her other X chromosome doesn’t have the faulty gene. Males aren’t so lucky here. If there’s a faulty gene on the X chromosome, they have only one copy, and that’s what they have to make do with.
This is why very few women have red-green colour-blindness, haemophilia or Duchenne muscular dystrophy. These are all disorders resulting from genes carried on the X chromosome, and in women they only show up if she has received a copy of that gene from both parents.
Something similar, but not quite the same, was happening with the obscure Russian fruit flies. The scientist made cross after cross, counting the offspring and finally solved the puzzle. It was the presence of a faulty gene on the X chromosome which caused the problem, but unlike the disorders I mentioned above, this gene was being passed on by the male flies. If a male fly had the faulty gene on his single X chromosome, it would affect his sperm. Exactly when the problem arose wasn’t clear, but the result was that he produced very few sperm with a Y chromosome, and therefore very few male offspring. However, he still made plenty of sperm with an X chromosome – all of which carried the faulty gene.
What so intrigued scientists about this gene was that the faulty gene was favoured by natural selection, and was likely to increase, but it was also likely to be harmful. It could, potentially, result in the extinction of the population if there were too few males. This was counter to what was understood about evolution at the time – where it was beneficial genes which were favoured by natural selection.
It wasn’t long before other examples of this kind of gene were found. They seemed to operate as a kind of gene parasite, looking after themselves at the expense of their hosts. Eventually, they became known as selfish genes or selfish genetic elements.
One selfish genetic element was discovered in red flour beetles in the early 1990s. This was found to have a different mechanism from any which had been discovered before. They named it Medea, which was an acronym, but also referenced the character from Greek mythology who murdered her own children, among others. If a female beetle had a copy of the Medea gene, then any of her offspring which lacked the Medea gene would die.
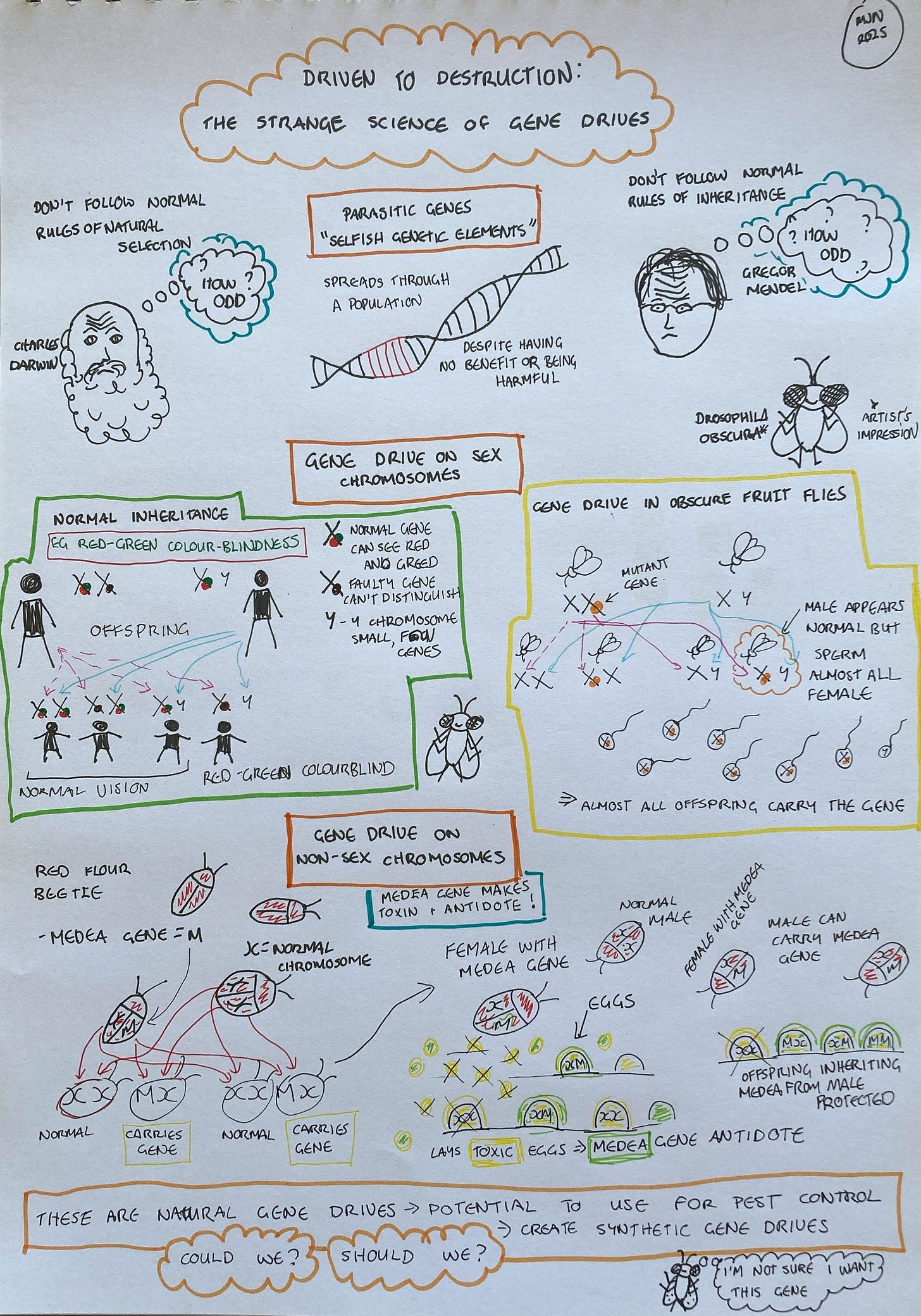
How this works isn’t obvious, so I’ll step back and explain in more detail. I mentioned earlier that our sex chromosomes are in pairs – for humans and fruit flies, either XX or XY. Our other chromosomes are in pairs as well, one of each pair from the mother and the other from the father. Sometimes, having two copies of our chromosomes is helpful, as we can cope with inheriting some kinds of faulty gene from one parent if the other is okay. But sometimes, having one copy of a faulty gene is a problem, even if the other is fine. Huntingdon’s disease, a severe neurological illness which typically strikes people between the ages of 30 and 50, is one such case.
The Medea gene is another example – having just a single copy results in the effect. But here’s where the Medea gene is really weird. Normally in a genetic disorder, it’s having the faulty gene which is harmful. In Medea, it’s the opposite. If a female beetle has the Medea gene, it’s her offspring without the Medea gene which die. If she mates with a male who also has the Medea gene, then some of her offspring which don’t get a Medea gene from her will get one from their father. These will survive. Those who don’t get the gene from either will only live for a few days.
How does this happen? This still isn’t entirely clear, but the current hypothesis is that it involves an inherited toxin and antidote. A female with the Medea gene produces a toxin in her eggs. The Medea gene also codes for an antidote, so the offspring which inherit that gene can overcome the toxin, while those without it die.
The Medea gene and the gene seen in obscure fruit flies are examples of what are known as gene drives. Scientists have been interested in the possibility of using gene technology to create them, as a potential means of controlling pests. The problem with using conventional gene editing techniques can be seen with the example of Douglas fir I wrote about a few weeks ago. Gene editing could produce sterile Douglas fir trees for use in plantations – these trees would not spread and become a problem. But this would do nothing to control the Douglas fir trees which are already wild and spreading in places they shouldn’t be.
But what if a gene drive could be used to spread a harmful gene through a pest population?
This is a possibility which has been getting much closer to reality in the last decade. Scientists have been working on a cousin of the fruit fly which has been spreading through Europe and the Americas, causing problems in berry and cherry crops. They’ve also been looking at whether such an approach could be used for mosquito control. As far as I can tell, these approaches are still experimental, but it sounds as if they are close.
The prospect of using gene drives raises some complex questions around risk. In the Douglas fir example, gene editing would render the trees sterile, which would mean the edited genes were not passed on. The risks of using the technology in this way are very small. A gene drive, however, would mean that the edited genes were intended to spread. In the case of the fruit fly I mentioned above, wiping it out in Europe and the Americas, where it has been recently introduced, seems like a good idea. But what if the gene drive spread back to Asia, where the fruit fly is native? Gene drives have been proposed as a possible future method for controlling pests like possums and stoats in New Zealand. But even if we could, does that mean we should? What if we controlled possums only for the gene to spread to Australia, where the possum is native?
In theory, a gene drive could be a powerful tool for pest control, but I also find the idea troubling. It’s not hard to think of plausible scenarios where a gene drive goes wrong, as opposed to some of the lower risk approaches to gene technology. It seems to be an approach which could be used for the wrong reasons, and have unforseen consequences. It also sounds frightening – it’s the kind of risk which prompts high outrage.
But I also know that any decision is not made in a vacuum. Our current approaches to managing pests can be expensive, harmful, or simply ineffective. We put toxins into the environment. We need to find people who are willing to carry bulky traps into remote areas, and then check them regularly. Our control of species like possums and stoats raises real issues around animal welfare. We are in an arms race with some pests, where as soon as we find something to control them, they develop resistance. Despite years of work, we still have hundreds of thousands of people a year dying from malaria.
We do need to consider new approaches, like gene drives. But we need to take a great deal of care with our decisions.
When I say these flies are obscure, I’m not kidding. Their scientific name is Drosophila obscura.
This is not the case for all insects and may be one reason fruit flies are so often used in genetic studies – the results are more relevant to us.
Love your illustrations!
And the way you interpret and present very complex topics.
Thanks for the effort you put into your articles.
After years of volunteer trapping I was excited by the prospect of gene drives to reduce the predator burden but Kim King dismissed that hope for me.